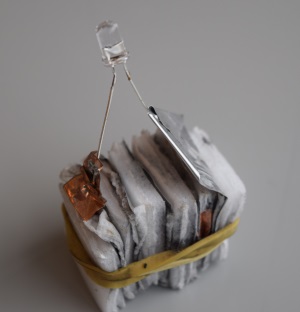
Aluminum Air Battery
Storing energy is one of the biggest challenges facing the scaling up of clean energy technologies. The goal of this activity is to allow students to design and build a battery using their understanding of oxidation and reduction reactions. Students will use everyday materials, including aluminum foil, salt water, charcoal, and copper foil, to build a non-rechargeable battery cell capable of powering an LED. Students should be familiar with balancing equations using half-reactions.
Essential Questions:
Can energy be captured and stored?
How can we capture the energy released from a chemical reaction to produce useful electrical power?
Background
Batteries store chemical potential energy which can be released to produce electrical energy, or electricity. Depending on the specific electrochemical reaction that takes place inside batteries, batteries may be either single-use (primary cell) or rechargeable (secondary cell). For example, cell phone batteries are rechargeable (e.g., we have to plug our phones in each night), however the batteries we put into our TV remotes and flashlights could be rechargeable and single use. In this experiment, we will learn about one kind of single-use battery, an aluminum air battery.
Aluminum air batteries use aluminum metal and the oxygen in the atmosphere as their electrodes. Aluminum air batteries offer one of the highest energy densities of all batteries because the weight of air is very light compared to other types of battery electrode materials. Energy densities are the amount of total energy output by a battery divided by the battery weight or the battery volume in units of Watt-hour/kilogram or Watt-hour/Liter. Energy densities are often used to evaluate the performance of a battery. Another set performance matrix that is commonly used together with energy densities is power densities, which is the amount of energy densities available every second of time (in units of Watt/kilogram and Watt/Liter). Aluminum air batteries are part of a larger category of batteries, metal air electrochemical batteries, wherein the pure metal forms the anode and the external air is the cathode. The batteries use the oxidation of aluminum at the anode and the reduction of oxygen at the cathode to form a galvanic cell. A galvanic cell generates electrical currents via spontaneous redox reactions in the cell. The aluminum air battery is a primary cell because the cell ingredients are consumed and the battery therefore cannot be recharged. The aluminum metal, Al, is completely reacted to produce aluminum hydroxide, Al(OH)3. The oxygen, O2, from air is reduced to produce hydroxide ions, OH–. Copper is used to collect the electrical current and is not consumed in the reaction. Crushed charcoal acts as a catalyst and greatly increases the surface area where the reactions can occur. The salt water solution is an electrolyte which carries the charged ions from one electrode to the other. The reaction rate can be increased by adding additional hydroxide ions using baking soda (NaHCO3) or strong base (NaOH or KOH).
- The anode oxidation half-reaction is Al + 3OH− → Al(OH)3 + 3e− −2.31 V.
- The cathode reduction half-reaction is O2 + 2H2O + 4e− → 4OH− +0.40 V.
- The balanced equation is 4Al + 3O2 + 6H2O → 4Al(OH)3 + 2.71 V.
(The reaction improves if it is done in a basic solution that supplies excess OH– ions. With potassium hydroxide electrolyte the 1.2 volts is produced with salt .7 volts per cell. Be very cautious if experimenting with KOH or NaOH electrolytes, use gloves and eye protection)
When a full circuit is formed with the aluminum air battery as shown in Figure 1 below, the redox reaction spontaneously begins due to the chemical potential difference between the two electrodes and forces the battery to discharge. Here the aluminum electrode is the anode of the cell since it hosts the oxidation half-reaction. Likewise, the air electrode is the cathode because it undergoes the reduction half reaction. During discharge, the electrons produced at the aluminum anode flow in the external circuit and power the load to which the circuit connects (see the purple solid arrows in Figure 1). A load in an electrical circuit is a component that consumes energy such as a lightbulb, a charging cell phone, or a working electric fan. At the same time, the negative OH− ions released by the air cathode travel across the liquid electrolyte and arrive at the aluminum anode to serve as reactants for the oxidation half-reaction (see the purple dashed arrow in Figure 1). The cycling of electrons and charged ions drives electric currents in the circuit, which powers the load in the circuit. Notably, when being charged, the flow of the electrons and OH− ions will be in the reversed direction of Figure 1.
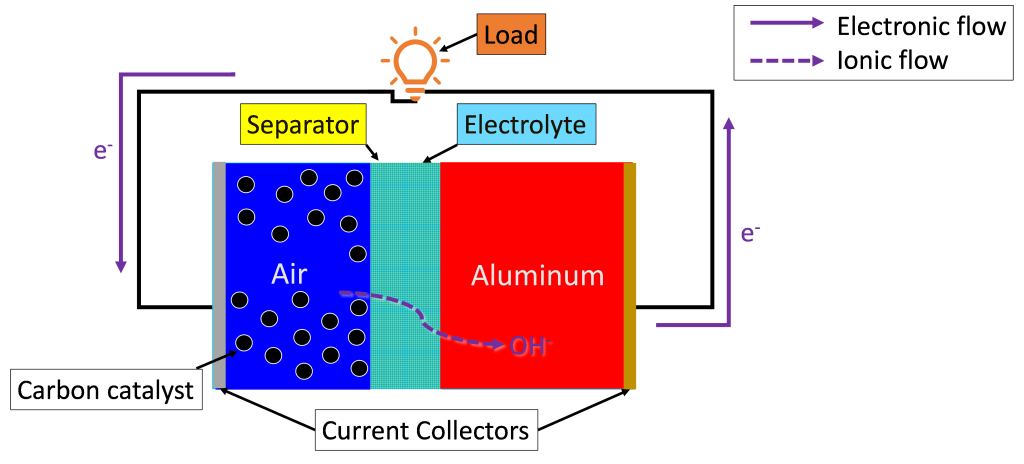
Figure 1. Diagram of a discharging aluminum air battery
In this lab, the students will learn how to build a structure which enables this electrochemical reaction to occur.
Research Connection
Researchers are trying to find new chemistries for high energy density batteries from earth abundant materials that are safe, reliable, and recyclable. While the specific aluminum air battery made in this lesson is not rechargeable, researchers are exploring new approaches to designing aluminum, and other metal air batteries that can be recharged. One important reason to optimize rechargeable batteries would be to store solar energy for use at night time. In the meantime, non-rechargeable metal air batteries are currently being investigated for aircraft, electric vehicles, and spacecraft because their high energy density makes them a long-lasting and relatively low-weight energy source. As seen in Figure 2, compared to Lithium-ion batteries, which are the most used battery chemistry for electric vehicles, and supercapacitors, which are applied to some hybrid-electric buses, metal air batteries have higher energy density. In fact, metal air batteries have energy densities that are most comparable to conventional combustion engines. However, metal air batteries show lower power densities than lithium-ion batteries, supercapacitors, and combustion engines.
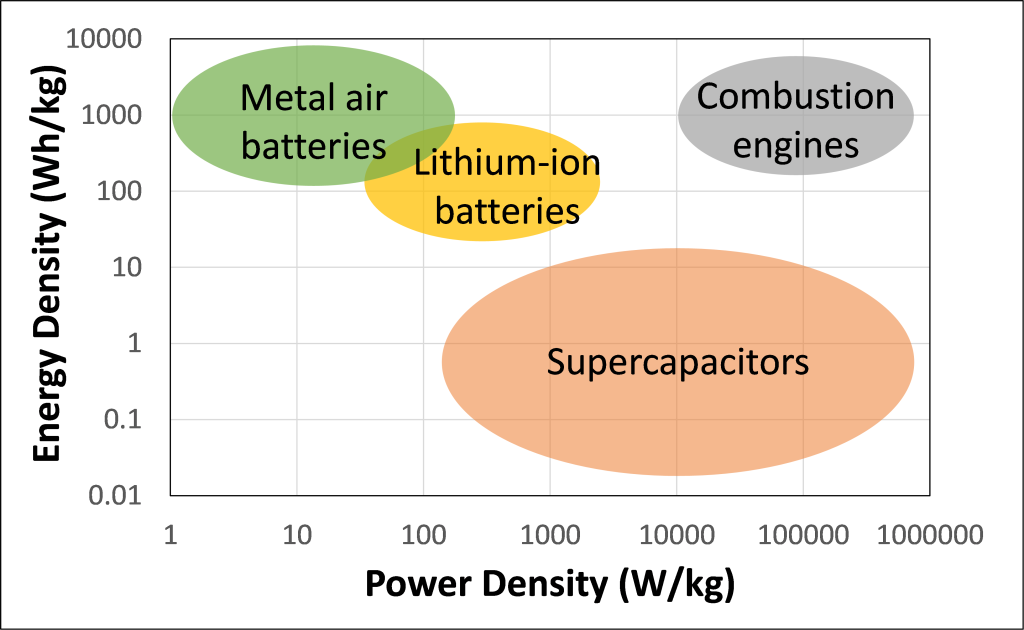
Figure 2. Energy density and power density of metal air batteries, Lithium-ion batteries, supercapacitors, and combustion engines. Data from Shao Y, El-Kady M F, Sun J, Li Y, Zhang Q, Zhu M, Wang H, Dunn B and Kaner R B 2018 “Design and mechanisms of asymmetric supercapacitors” Chem. Rev. 118 9233–80
NGSS Standards
Standard Number | Standard text |
HS-PS1-2 | Construct and revise an explanation for the outcome of a simple chemical reaction based on the outermost electron states of atoms, trends in the periodic table, and knowledge of the patterns of chemical properties. |
HS-PS1-5 | Apply scientific principles and evidence to provide an explanation about the effects of changing the temperature or concentration of the reacting particles on the rate at which a reaction occurs. |
HS-PS3-3 | Design, build, and refine a device that works within given constraints to convert one form of energy into another form of energy. |
Safety
Fine charcoal/graphite powder can cause eye irritation, skin irritation, gastrointestinal irritation, and respiratory tract irritation when contacted, consumed, or breathed in. While the amount of chemicals used in the lesson are not dangerous, eye protection, mask, and chemical-resistant gloves (e.g., latex, Nitrile) should be worn while working with chemicals. Always maintain a good distance between your nose and the charcoal/graphite powder.
Materials/Preparation
- 1 small cup (e.g., 8 oz mason jars work well)
- Salt water mixture (dissolve approx. 1 tbsp salt in 1 cup water)
- Aluminum foil (approx. 12 inch square)
- Paper towel (approx. 12 inch)
- Any type of carbon powder such as Charcoal briquette, graphite powder, or activated charcoal ground to a coarse powder. The texture of the charcoal should at largest be that of coarse corn meal. If you cannot find pre-ground charcoal, you can wrap the charcoal in paper and pound with a hammer to shatter the lumps.
- Copper foil tape with conductive adhesive or unvarnished copper wire (approx. 12 inch strip)
- Two wires with alligator clips
- Multimeter and / or LEDs (to represent the load)
- Scissors (If aluminum foil needs to be cut)
- Print out the Aluminum air battery student worksheet, 1 per student
- Optional: scotch tape
Introduction
- Have the students balance the half reactions and form the complete balanced equations listed in the Background. (See student worksheet step #1)
- Check the equations as a class and discuss the type of reaction this represents. Discuss the connections between the redox reactions and the cathode/anode terminologies in Figure 1, i.e. during discharge, the cathode is reduced and the anode is oxidized. (See student worksheet step #2)
- Based on the above discussion, have students assign each half reaction to the cathode and the anode in the aluminum air battery student worksheet step #3 and determine the directions of the electron flow and the ionic flow in the Aluminum-air battery. (See student worksheet step #3)
- Tell students that today they will be building a battery.
Main Activity
- Show the students the materials they will be using to build the aluminum air battery (e.g., aluminum foil, copper foil, etc.) and have them guess the roles of each material in the battery cell. (See student worksheet step #4)
- cathode = oxygen in the air
- cathode activator/catalyst = carbon
- separator = paper towel
- anode = aluminum foil
- current collector = copper foil/wire
- electrolyte = salt water
- load = LED/multimeter
- Hand out materials to students and give them time to explore the properties of the material before they are connected. Students can use a multimeter to measure the voltage and current of the materials. Some conclusions they might draw are: the paper towel is porous and allows liquid to soak in, the aluminum and copper foils are the most heavy components, etc.
- Once students have had time to explore the materials, tell them that their next challenge is to use these materials to construct an aluminum air battery that will exhibit a voltage. Encourage them to use the diagram, the equations, and their explorations to build. (See student worksheet step #5) The standard procedure for making the aluminum air battery is as follows:
- Gather a 6-inch square of aluminum.
- Put a 6-inch square of paper towel on top of the aluminum.
- Offset the position of the paper towel 1-2 inches from the aluminum. The overhanging towel prevents a connection between the copper and the aluminum.
- Add approximately ½ tablespoon of carbon powder to the center of the paper towel.
- Place the copper strip in the center of the mound and extend it 2 inches past the aluminum. Make sure the copper wire does not make contact with the aluminum. See the example Figure 3 below.
- Mix salt and water in the cup and drip the saturated salt water onto the carbon powder until the paper towel is fully wet.
- Now we are ready to test the battery!
Figure 3. Example of a completed aluminum air battery
- Connect each alligator clip to each probe of the multimeter. Using the alligator clips, connect the other positive end of the multimeter to the outstretched copper strip and the negative end of the multimeter to any part of the aluminum foil. (See student worksheet step #6)
- Connect the battery to an LED. Does the LED light up? Why or why not? (See student worksheet step #7)
- Repeat steps 1-6 to create multiple battery cells. Wire the cell to the existing cell in series and measure the voltage of the chain. What voltage do you see? Is it enough to light the LED? (See student worksheet step #8)
- Connect cells to the chain one-by-one until there is enough voltage to light the LED. LEDs usually require 2-3 volts of voltage to power. What is the minimum voltage and/or current required to light your LED? (See student worksheet step #9)
- For an extended discussion on Tesla Model S fire in 2013, see student worksheet step #10 and #11)
Summary
Use one or more of the following questions with students to help them debrief the activity and demonstrate what they have learned.
- What design requirements must be considered when designing a battery that uses these reactions? (E.g., supply of oxygen, evaporation of electrolyte, maintenance, surface area considerations, copper connections between cells, inclusion of charcoal as a catalyst or conductor.)
- What voltage did you achieve?
- How did the voltage vary with time?
- What is the minimum voltage it takes to light an LED?
- How can you increase the availability of oxygen to the cell?
- Is there a limit to the voltage the chained batteries can achieve?
- Is it possible to “wake up” a cell after a few days if it stops producing?
- What would be the advantage to using a flowing or circulating electrolyte?
- Why was the voltage you achieved different from our theoretical prediction from the balanced equations?
- How are battery technologies related to clean energy?
Extensions
To help students further explore these concepts, consider the following extensions.
- Try other electrolyte solutions. (e.g., vary the salt to water ratio of your solution, try adding a bit (1 tsp – 1 tbsp) of different soaps and vinegar). How does this affect the voltage of the cell?
- Reverse the design of the battery by layering the charcoal power, copper foil tape, separator, and electrolyte first, and then cover everything with a layer of the Aluminum foil. Measure the voltage and current again. What changes did you experience with this “face-down” battery?
- Vary the coarseness of the carbon powder. What coarseness works the best? Why?
Resources and References
- Aluminum air battery Wikipedia
- An Open-Ended Project: Building a High Performance, yet Simple, Household Batteryby Ping Y. Furlan, Thomas Krupa, Humza Naqiv, and Kyle Anderson. Journal of Chemical Education 2013 90 (10), 1341-1345
- Fostering Innovation through an Active Learning Activity Inspired by the Baghdad Battery by Xu Lu and Franklin Anariba. Journal of Chemical Education 2014 91 (11), 1929-1933
- Aluminum—Air Battery by Modesto Tamez and Julie H. Yu. Journal of Chemical Education 2007 84 (12), 1936A
- The Salty Science of the Aluminum-Air Battery by Stephanie V. ChasteenUniversity, N. Dennis Chasteen, and Paul Doherty. The Physics Teacher. 2008 46 (9), 544
- Metal air battery: A sustainable and low cost material for energy storage by Deepti Ahuja, Varshney Kalpna, and Pradeep K Varshney 2021 J. Phys.: Conf. Ser. 1913 012065
- The 2021 battery technology roadmap by Jianmin Ma et al 2021 J. Phys. D: Appl. Phys. 54 183001