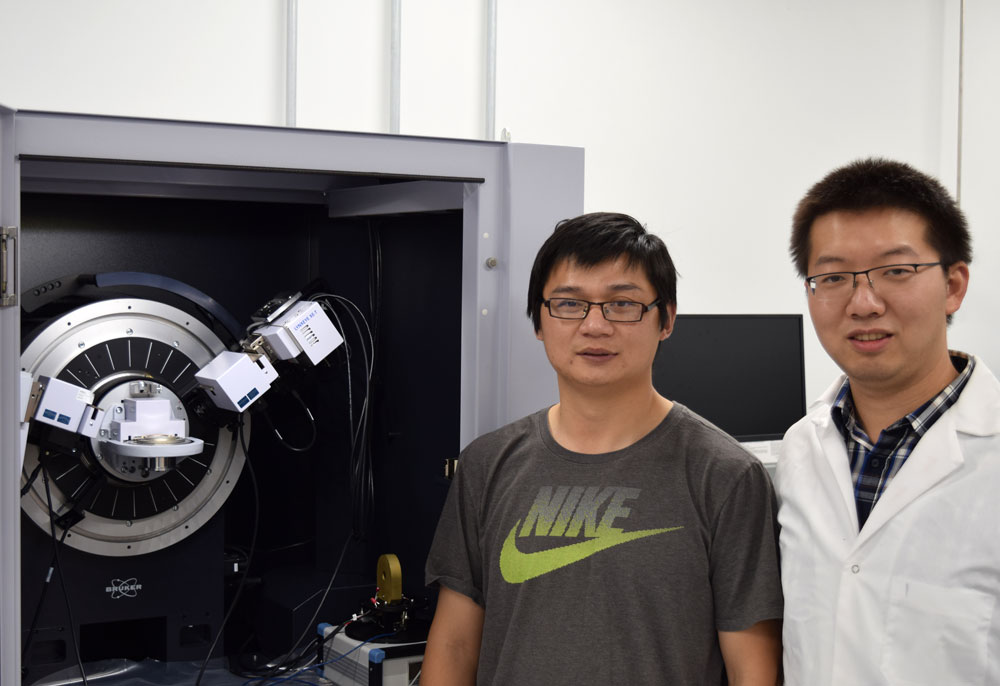
Shanyu Wang (left) and Mengyu Yan (right), pictured with the x-ray diffractometer (XRD) located at CEI’s Washington Clean Energy Testbeds. Photo: Owen Freed / University of Washington
UW materials scientists make breakthrough in study of alternative to lithium-ion
January 23, 2018
The lithium-ion batteries found in smartphones, power tools, and electric cars are small and lightweight, but the technology is generally considered to be infeasible for energy storage at a much larger scale. The typical cathode material (lithium cobalt oxide) is expensive and susceptible to overheating, and the electrolyte (dimethyl carbonate) is highly flammable. If these batteries are overcharged or are physically damaged, there can be explosive consequences. To circumvent these issues and develop batteries that can store energy at the utility scale, battery researchers have been focusing on models that use earth-abundant elements and water-based electrolytes.
University of Washington materials science & engineering (MSE) researchers have made a breakthrough in understanding the mechanics of a zinc-ion, aqueous-electrolyte model. This alternative technology is lower in energy density than lithium-ion batteries, with 30 times the power density. Jihui Yang, the Kyocera associate professor of MSE and MSE department chair, said that the research “points to a high-performance, low-cost, safe, and environmentally-friendly battery, ideal for grid energy storage.”
Mengyu Yan, a Washington Research Foundation (WRF) Innovation Postdoctoral Fellow and the lead author of the study published in Advanced Materials, and Shanyu Wang, also a postdoctoral researcher in Yang’s group, created the cathode by growing vanadium pentoxide (V2O5) nanowires on graphene.
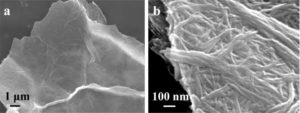
Scanning-mode electron microscope image of bare graphene sheets (a) and as-prepared V2O5 nanowires, after liquid-phase deposition onto the graphene (b)
The resulting vanadium oxide-graphene (VOG) material was found to contain water molecules within the layers of the structure, like the frosting of a layer cake.
The existence of these water molecules is key to optimizing the mechanism of charge and discharge.
During charge and discharge, zinc ions intercalate between the VOG cathode layers. Intercalation – reversible insertion and de-insertion, through chemical processes – is a vital design element of the majority of rechargeable battery technologies, including the lithium-ion model. The major finding of the UW researchers was a curious “lubricating” phenomenon that takes place during intercalation. The aforementioned water molecules found within the VOG increase interlayer distance, and also form weakly-bonded “shields” around the zinc ions, reducing their effective charges and thus their attraction to oxygen atoms within VOG crystals.
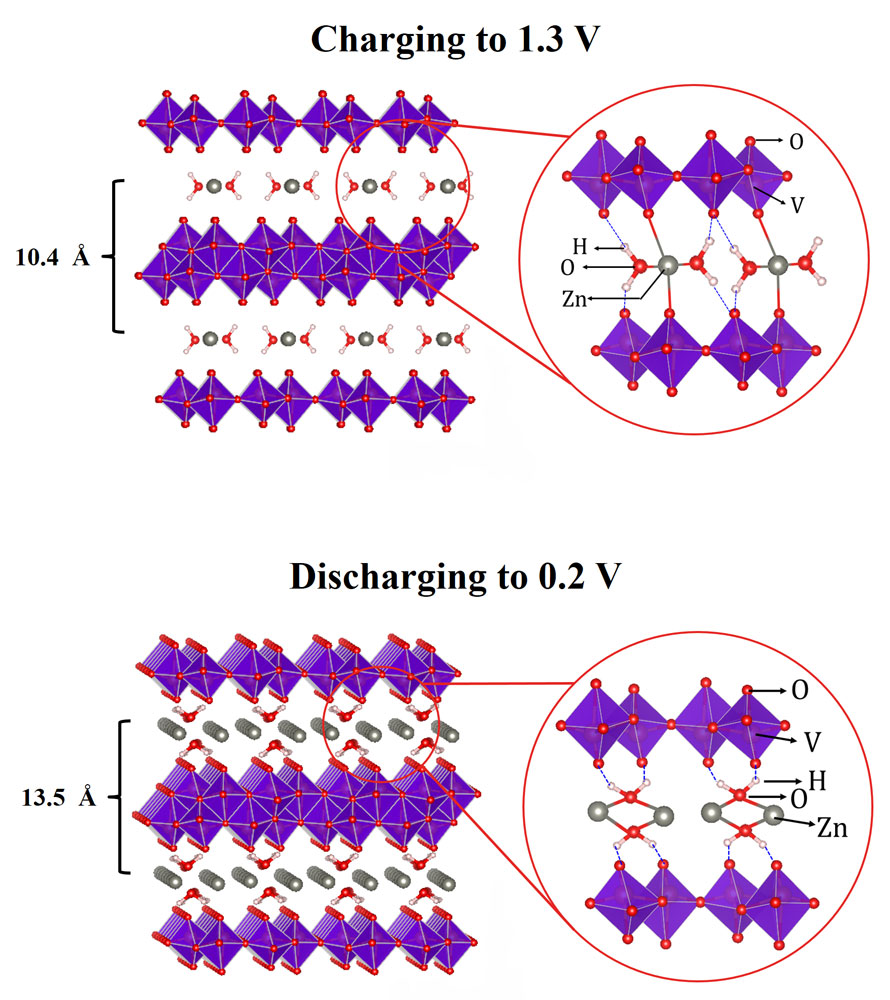
Artist’s representation depicting interlayer gap and shielding effects of water molecules during Zn intercalation
When the structural water molecules were removed by annealing VOG at 350°C, both peak performance and performance over multiple cycles dropped significantly. High rates of intercalation result in faster power transfer and longer battery lifetimes, which are primary concerns of grid-scale battery engineers.
The research benefited from a partnership with the Pacific Northwest National Laboratory (PNNL), located in Richland, WA. PNNL is a leading member of Battery500, a U.S. Department of Energy (DOE) program that involves multiple national labs and research universities, which lead author Yan said was vital to the project:
“Battery500 members shared important information about the latest battery technologies and their potential uses. In this case, zinc-based models were promising for grid-scale use. As well, we were able to rely on the advanced NMR [nuclear magnetic resonance] instrumentation and expert technicians at PNNL to help us collect and interpret data.”
Both Yan and Wang feel optimistic about the future of the technology, but highlighted different areas for advancement. Wang shared his views on the developments needed to get a zinc-ion battery on the market:
“We now understand the physics of this model, so we’re excited about applying our understanding of water’s role in intercalation to other types of battery technologies. While vanadium is cheap, it’s relatively toxic, so we will try different materials like manganese oxide. We could also try different polar solvents instead of water, to try and further improve efficiency. Graphene is a great material for studying [the intercalation mechanism], but it makes this particular prototype too expensive for a grid scale, so we need to explore a different material.”
Yan was excited about the potential for “further cooperation with PNNL to reduce the price, such that this technology could be implemented for grid-scale energy storage.”
Along with the NMR data collected at PNNL, the researchers utilized CEI’s Washington Clean Energy Testbeds to perform x-ray diffraction (XRD), cyclic voltammetry (CV), and scanning-mode electron microscopy (SEM). The characterization-focused facility allowed Yan and Wang to collect information about the crystal structure and electrochemical properties of their device.
Yan earned his Ph.D. in materials science and technology at Wuhan University of Technology (Wuhan, China), where he was supervised by Liqiang Mai, the chair professor of materials science. Their relationship has continued to be fruitful, as Mai and his research group contributed to discussions of the particulars of the intercalation model, analysis of electrochemical data (cyclic voltammetry, energy density, and power density), and authorship of the Advanced Materials paper.
Co-authors of the paper include Dr. Ying Chen, Dr. Yuyan Shao, Dr. Karl. T. Mueller, and Dr. Jun Liu at Pacific Northwest National Laboratory; Pan He, Dr. Qiulong Wei, Kangning Zhao, Dr. Xu Xu, Prof. Qinyou An, Yi Shuang, and Prof. Liqiang Mai of Wuhan University of Technology.
For more information, contact Dr. Yan at myyan@uw.edu and Professor Yang at jihuiy@uw.edu.