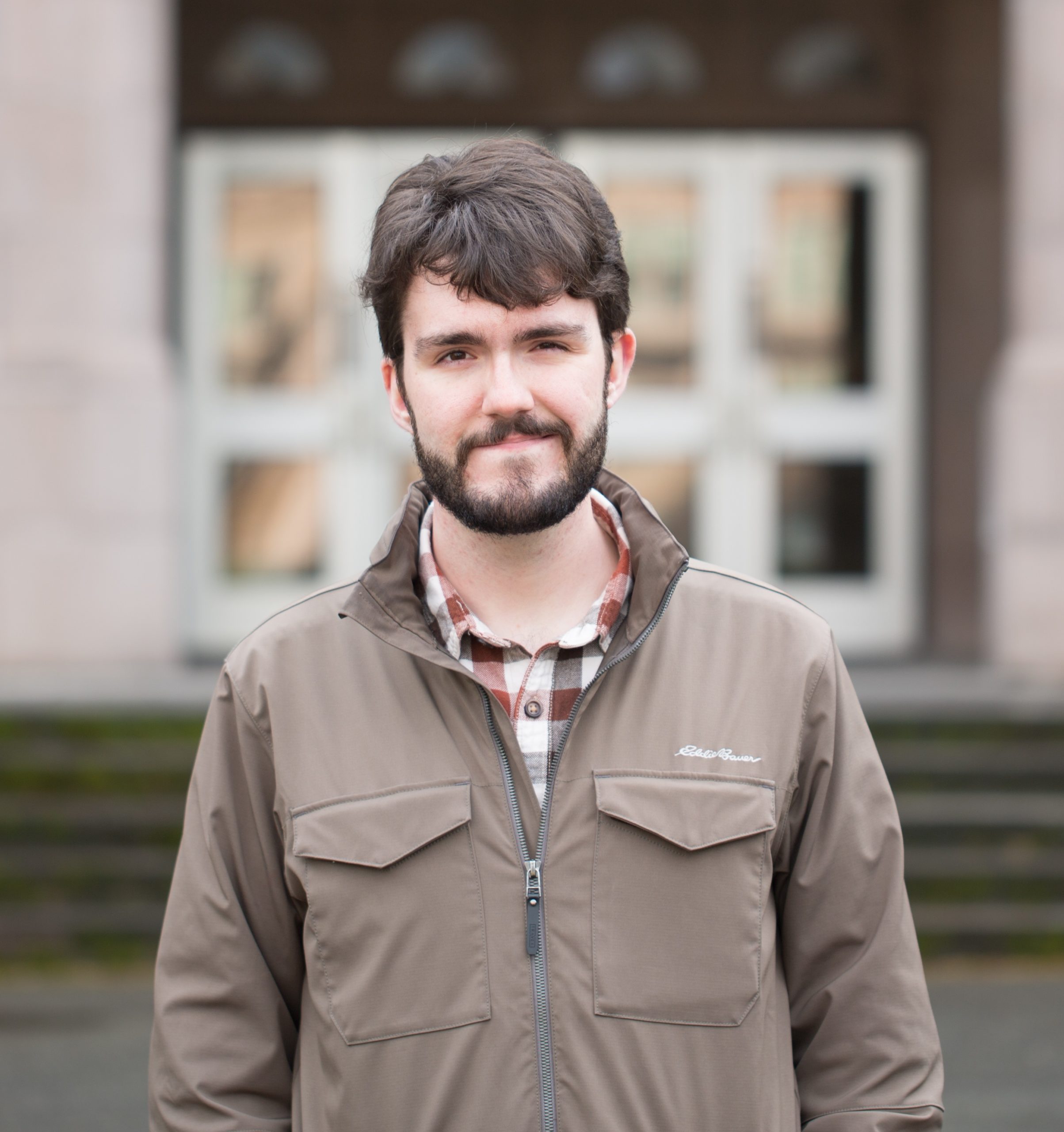
2015-16
Wide-scale adoption of clean energy sources will require batteries with better energy density and high resource availability. Silicon-nanoparticle-based lithium-ion batteries fit these criteria, as silicon can theoretically store up to ten times the charge of typical battery anode materials. However, silicon-based anodes have yet to reach commercial maturity due to silicon’s tendency to expand and contract wildly during battery operation. This volume change process disrupts the battery microstructure, consisting of conductive carbon and inert polymer binder, and causes the battery to fail after only a few uses.
Multifunctional conducting polymers have been demonstrated as effective additives to circumvent this problem, since they can be designed to maintain both conductivity and mechanical integrity of the anode during the silicon volume change process. This exciting approach has only been recently proven as a robust solution, and many hurdles remain before such conducting binders become commercially viable. My research attacks these issues from both a device optimization standpoint and a chemistry standpoint, connecting molecular design to crucial battery performance parameters such as cycle life and volumetric capacity. With a combination of novel approaches and detailed characterization, I plan to make the silicon anode a realistic solution to our energy storage problems.
2014-15
As global society continues to grow into the 21st century, we find ourselves in the midst of an energy paradox, needing to both minimize the environmental impact of our energy use, yet requiring more of it than ever, in more places. Meeting both of these needs simultaneously will require energy storage technology that provides longer lifetime, better power density, and higher resource availability than the current gold standard: the lithium-ion battery. It’s no secret that this technology has evolved at a much slower pace than our needs over the past two decades. Materials and ideas already exist, specifically the silicon nanoparticle anode, that could boost lithium-ion performance to the point of a breakthrough. However, this technology has yet to be fully realized because of silicon’s tendency to expand and contract wildly during battery use, leading to a host of internal problems. Recently, a variety of novel polymer systems have been demonstrated to nicely mitigate the undesirable effects of this volume change, but their structure, synthesis and mechanism of action have yet to be fully explored and optimized. To this end, my goal is to design, characterize, and implement new conducting polymer binders specifically for use in batteries. By combining rational molecular design principles with insight into system-level behavior, I am working to create a polymer additive that would enable anodes based on energy-dense, long-lasting, Earth-abundant silicon. This research should go a long way towards meeting society’s energy storage needs going forward, in terms of both sustainability and portability.
Advisor: Alex Jen, Molecular Engineering & Sciences